The QCLAM is one of two magnet spectrometers at the S- DALINAC. It is used for (e,e') and (e,e'x) experiments at various incident beam energies and scattering angles. Electrons which have been scattered from a target nucleus will enter the spectrometer and be deflected by the pentapole and dipole magnet according to their respective impulse. These electrons can then be detected by the position sensitive wire chambers and their energy and scattering angle can be reconstructed.
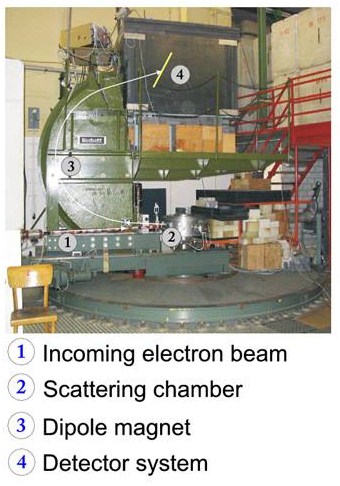
Electron scattering is an essential tool for nuclear structure studies. The height energy resolution is very important in order to resolve nuclear transitions specific interest.
The 169° spectrometer with its beam transport system is used for high-resolution electron scattering experiments and can be can be operate in a conventional and “energy-loss” mode which allows to perform (e,e´) experiments independent on the energy of the electron beam.
The electron scattering experiments at S-DALINAC have been successfully realized for many years. A new focal plane detector system based on modern silicon microstrip detectors together with fast readout electronics and a new radiation shielding have been developed. The main advantages of the new detector system are the ability to process very high count rates, a high spatial resolution and an easy mechanical setup.
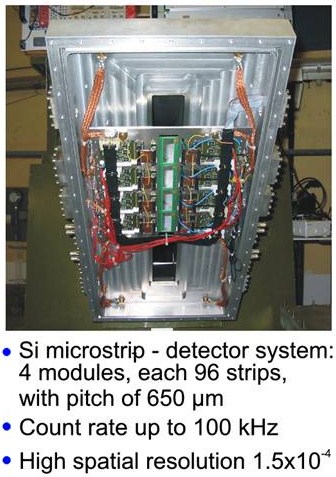
The following 94Mo spectrum is an example for the measured spectra using the Lintott spectrometer in dispersion-matching mode. It was measured at an incident electron beam energy and beam currents of. The energy resolution in this spectrum was about.
Reaction rates for photon induced nuclear reactions are measured in various experimental setups for the study of nuclear structure.
Different facilities offer different advantages, while most lack a good or even any resolution for the excitation energy.
NEPTUN is capable to measure reaction rates as a function of excitation energy with the help of tagged photons. The energy resolution of 50 keV and better is excellent.
The photons are produced by the effect of bremsstrahlung. The monoenergetic electrons from the S-DALINAC impinge onto a thin radiator target made from gold. Here, bremsstrahlung is induced. The thickness of the radiator target is chosen accordingly to let the electrons produce at most one photon. The scattered electrons are analyzed by a large dipole magnet. They are focussed to different positions according to their momentum in the focal plane of the magnet. An array of detectors with a high spatial granularity detects the electrons and therefore determines their energy.
The related photons continue to the experimental target and can induce nuclear reactions. The reaction products are detected by corresponding detector arrays. By making use of the time relation, the induced reaction products are assigned to the related electron.
The energy of the photon which induced the reaction can easily be calculated as the difference of the incoming electron beam energy minus the scattered electron's energy.
For the measurement of (g,n) reaction rates a neutron detector array is in operation.
For the measurement of (g,g') reaction rates a LaBr3 scintillator array is currently under construction.
For both arrays we pursue a digital data acquisition, which is being developed at the moment.
NEPTUN is also well suited for the characterization of gamma detectors. It can be considered as a monoenergetic gamma source with tunable intensity and energy. The beam current can be adjusted to arbitrarily low values.
We always look for motivated students to reinforce our team! If you are interested, please contact us for a tour and information without obligation.
Previous campaigns:
extended detector characterization campaign including the materials HPGe, LaBr3 and CsI:(Tl) . A prototype detector for the CALIFA calorimeter barrel has been investigated, which will be used at the future FAIR facility.
The Darmstadt High Intensity Photon Setup (DHIPS, K. Sonnabend et al., Nucl. Instr. and Meth. A 640 (2011) 6) is situated right after the injector of the S-DALINAC electron accelerator. The incident 10 MeV electron beam is produced by the injector of the S-DALINAC. A schematical overview of this setup is presented in the Figure. Photons are generated as bremsstrahlung from electrons bombarding a massive radiator target. The originated photons pass a copper collimator and hits at a first target position a scattering sample. The sample is surrounded by three High Purity Germanium detectors equipped with active Anti-Compton shielding. At a second target position polarization measurements are performed using a Compton Polarimeter. The whole setup is shielded by lead.
Compton tracking polarimetry using a highly segmented HPGe detector of the AGATA type
Photon scattering experiments are performed in Darmstadt at the DHIP-setup. In these experiments mainly magnetic and electric dipole excitations are observed by measuring de-exciting gamma-rays with HPGe detectors. For an unambiguous scientific interpretation, e.g. in the context of the pygmy dipole response, the electric excitation mode has to be distinguished from the magnetic one. Since the parity of the ground is state is known, therewith the parity of the corresponding excited nuclear state is measured by observing the linear polarization state of the depopulating gamma-rays (~4-10 MeV) of the nuclear level of interest.
The method of Compton polarimetry to determine the linear polarization of gamma-rays uses the angle-dependence of the Compton scattering process on the orientation of the electric field vector of the incident photons, according to the Klein-Nishina cross-section. Electric multipole radiation can be distinguished from magnetic radiation by measuring the according characteristic asymmetry-curve of occuring Compton scattering angles.
A conventional technique is to use a gamma-ray polarimeter consisting out of one central detector serving as a Compton scatterer and a pair of detectors, absorbing the scattered photons. The latter detect the scattered photons typically at angles phi = 0° and phi = 90° with respect to the plane defined by the photon beam direction and its polarization orientation.
Such a setup can reach maximum polarization sensitivity at the expense of detection efficiency due to insignificant solid angle coverage of the analyzing detectors. Efficiency can be increased by converging scattering and analyzing detectors, but then the resolution of the Compton scattering angle is low. Here the concept of polarimetry using a highly segmented, and therefore position sensitive detector becomes obvious: by measuring precisely the location of gamma-ray interaction points, Compton scattering angles can be observed continously.
Important quantities to describe the quality of the polarimeter are the polarization sensitivity and the figure of merit, which includes the detection efficiency as well.
With this concept the DArmstadt GAmma ray Tracking Assembly DAGATA was funded within the collaborative research center SFB 634 to measure the polarization of high energetic gamma-rays, where the analyzing power of the Compton effect is too low for the use of conventional Compton polarimeters.
Currently one detector of the AGATA-type is mounted within a triple-cryostate, the other two detectors are funded and ordered.
In a calibration measurement we were able to prove that the polarization sensitivity of the one-detector DAGATA is significantly larger in comparison with conventional four-fold polarimeters.
Details can be found in the according publication:
B.Alikhani, et al,
NIM A 675(2012)144
http://www.sciencedirect.com/science/article/pii/S016890021200174X
Next steps of the project are:
- to implement the other two detectors in DAGATA
- to repeat the calibration measurement with the full triple detector
- to integrate the detector within the NRF setup DHIPS
- to use pulse shape analysis and improved gamma-ray tracking techniques
- to perform first in-beam measurements.